Transformer sizing involves selecting the appropriate kVA rating, which represents the transformer’s total capacity. The kVA rating is determined by factors such as voltage, current, frequency, temperature, and load requirements.
To choose the right transformer size, consider the specific application, environment, and whether a single-phase or three-phase transformer is needed. Dry-type or liquid-filled transformers with different cooling methods are available to suit various requirements.
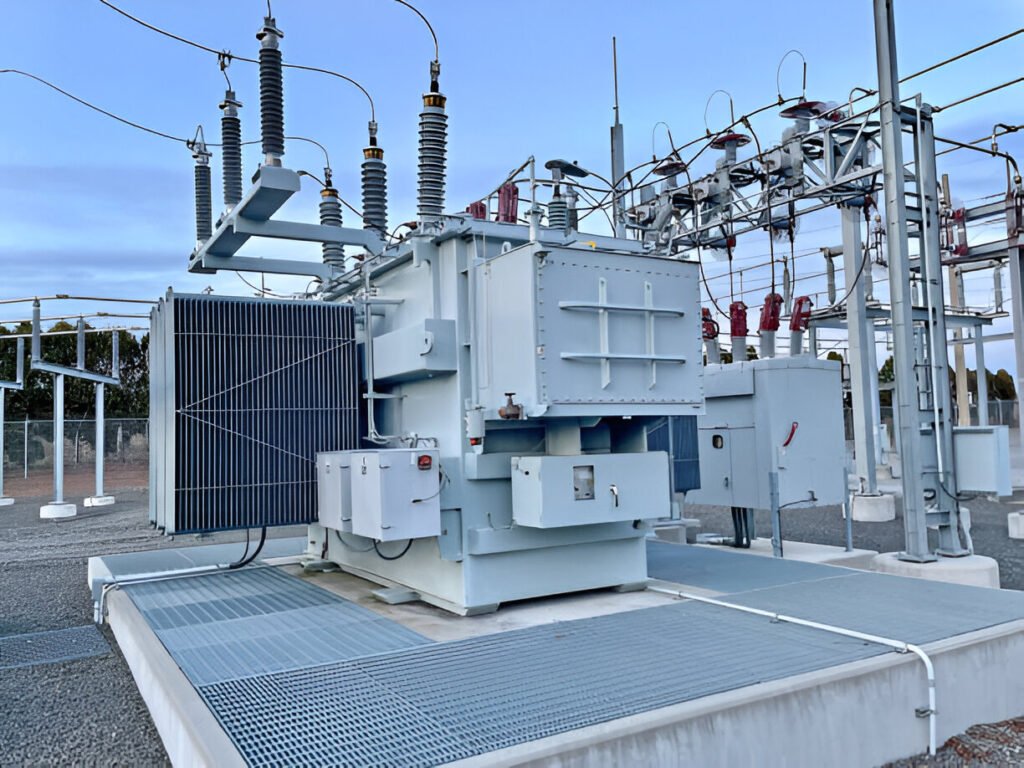
Understanding kVA Ratings
Definition of kVA (kilovolt-ampere) and how it differs from kW (kilowatt)
kVA (kilovolt-ampere) measures apparent power, which includes both real power (kW) and reactive power (kVAR). Real power performs actual work, while reactive power is temporarily stored in the magnetic fields of inductive loads like motors and transformers.
To calculate kVA, multiply voltage by current and divide by 1,000. A transformer with 480V and 100A has a kVA rating of 48 (480 x 100 / 1,000). kW is calculated by multiplying voltage, current, and power factor (PF), the ratio of real power to apparent power (0 to 1). A transformer with 480V, 100A, and a power factor of 0.8 has a kW rating of 38.4 (480 x 100 x 0.8 / 1,000).
Formulas for calculating kVA ratings
Single-phase transformer kVA rating formula: kVA = (Volts x Amps) / 1,000. Multiply voltage and current, then divide by 1,000.
Three-phase transformer kVA rating formula: kVA = (Volts x Amps x 1.732) / 1,000. Multiply voltage, current, and 1.732, then divide by 1,000. The constant 1.732 accounts for the phase difference in three-phase systems.
Why transformers are rated in kVA instead of kW
Transformer ratings are expressed in kVA (kilovolt-amperes) instead of kW (kilowatts) because kVA represents the apparent power. Apparent power takes into account both the resistive (real) and reactive components of the load, while kW only represents the real power consumed by the load.
Transformers must be sized to handle the total apparent power, including any reactive loads such as motors, capacitors, or inductors. Reactive loads can cause the current to be out of phase with the voltage, resulting in a power factor less than 1. The kVA rating ensures the transformer can handle the total current flowing through it, regardless of the power factor.
Using kW ratings for transformers could lead to undersizing if the load has a significant reactive component. Undersizing could cause overheating and damage to the transformer. kVA ratings ensure the transformer is adequately sized to handle the total apparent power demand of the load. This provides a safety margin and prolongs the life of the transformer.

Standard Transformer Sizes and Ratings
Overview of standard kVA ratings for single-phase and three-phase transformers
Single-phase transformer standard kVA ratings typically include 5, 10, 15, 25, 37.5, 50, 75, 100, 167, and 250 kVA. These ratings accommodate a broad spectrum of power needs for residential, commercial, and industrial single-phase applications.
Three-phase transformer standard kVA ratings commonly include 30, 45, 75, 112.5, 150, 225, 300, 500, 750, 1000, 1500, 2000, and 2500 kVA. Commercial and industrial settings with higher power demands often utilize three-phase transformers.
Typical voltage ratings and configurations
Single-phase transformers commonly have primary voltages of 480V, 240V, or 120V, while secondary voltages are often 120V for residential and light commercial applications. Three-phase transformers, typically used in commercial and industrial settings, may have primary voltages of 480V, 600V, or 4160V, with secondary voltages determined by the specific equipment needs.
A standard configuration for a three-phase transformer in a commercial building is a 480V primary and a 208Y/120V secondary. This setup provides 208V for powering larger equipment and machinery, while the 120V is used for lighting, receptacles, and smaller appliances. The “Y” in 208Y/120V refers to the wye configuration, which is a common method of connecting three-phase transformers and loads.

What affects the sizes and ratings of the transformer
Voltage
Transformers with higher voltages have smaller physical dimensions than lower voltage transformers of the same kVA rating. Higher voltages allow for lower currents, enabling the use of smaller wire sizes in the windings. However, higher voltage transformers require enhanced insulation and larger clearances to prevent electrical breakdowns.
Lower voltage transformers require larger wire sizes to accommodate higher currents. This results in increased winding sizes and overall transformer dimensions. Low voltage applications typically demand transformers with higher kVA ratings to handle the increased current requirements.
Current
The amount of current flowing through the transformer’s windings is a major factor in determining its size and capacity. Larger cross-sectional areas for the windings are required to accommodate higher currents without overheating or causing excessive voltage drops.
The maximum expected load current and any potential short-circuit currents must be considered when selecting a transformer. These currents determine the appropriate wire gauge and insulation requirements for the windings. The current density in the windings should be kept within acceptable limits to prevent overheating and ensure efficient operation.
Power
Transformer kVA ratings must exceed the total load power, providing a safety margin to handle temporary overloads and extend the transformer’s lifespan. Oversizing allows the transformer to operate reliably without being overstressed. However, excessive oversizing leads to inefficiency and higher costs.
Load types influence the transformer’s power rating. Resistive loads, such as heating elements, have a power factor close to 1. Inductive loads, like motors, have lower power factors. For inductive loads, the transformer’s kVA rating must be higher to supply the required active and reactive power components.
Frequency
Transformer frequency, either 50 Hz or 60 Hz, significantly impacts the size and kVA rating of the transformer. Higher frequency transformers are generally smaller and have lower kVA ratings than their lower frequency counterparts. Higher frequencies enable more efficient magnetic flux transfer in the transformer core, reducing the required cross-sectional area of the core and the number of winding turns.
Higher frequencies also result in increased core losses, including eddy current and hysteresis losses. Thinner laminations or higher-grade core materials may be necessary to mitigate these losses, which can affect the overall cost of the transformer.
Temperature
As the ambient temperature increases, the transformer’s heat dissipation capability decreases, resulting in higher operating temperatures. Lower ambient temperatures enable better heat dissipation, allowing the transformer to operate at lower temperatures.
Efficiency
Select a transformer that operates at peak efficiency under typical load conditions. Transformer efficiency is highest when the load is between 50-100% of the rated capacity. Operating consistently below 50% load reduces efficiency and can cause overheating due to higher core losses.
Load Requirement
Calculate the total kVA of all loads the transformer will supply, considering both present and future requirements. Factor in load diversity, as not all connected loads draw their maximum current simultaneously. Apply appropriate demand factors based on load type, such as lighting, motor, or heating.
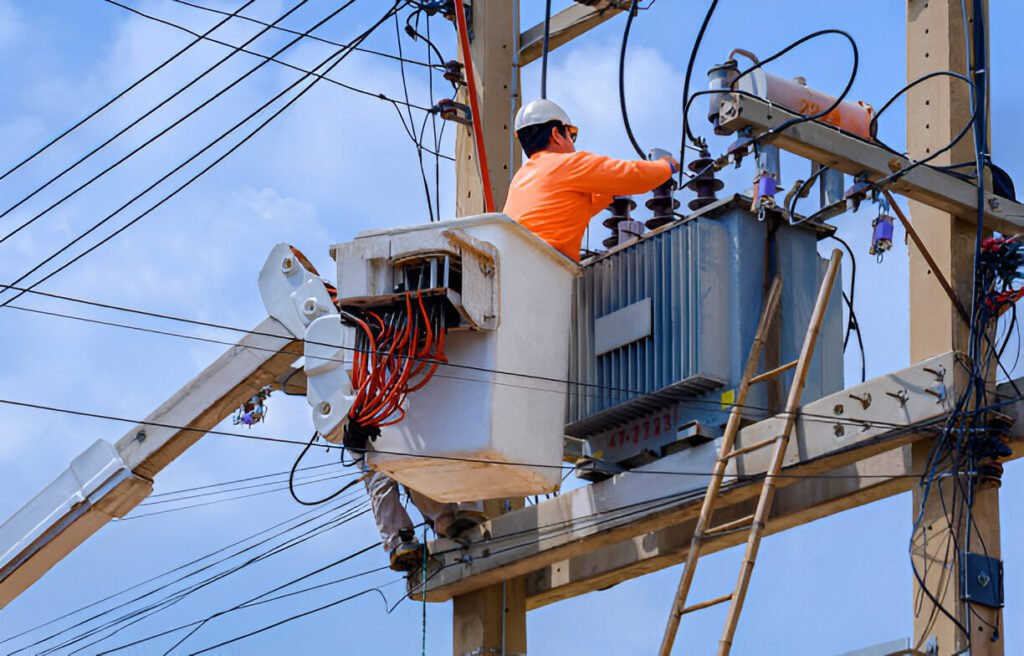
Factors to Consider When Sizing a Transformer
Calculate Electrical Load
Add up the wattage ratings of all devices and equipment that will be connected to the transformer. Alternatively, measure the current draw and voltage of the entire system. Include both continuous and intermittent loads in the calculation.
After determining the total load, add a safety margin of 20-25%. This safety margin prevents overheating and premature failure of the transformer by allowing it to handle unexpected surges or overloads.
Determine Supply Voltage
To determine the appropriate supply voltage, first identify the available voltage sources in your facility, such as 120V, 240V, or 480V. Next, check the voltage requirements of the equipment or load that will be powered by the transformer.
The transformer’s primary voltage rating must match the supply voltage to prevent damage or inefficient operation. Mismatching the supply voltage and transformer rating can result in incorrect output voltages, overheating, or transformer failure.
The supply voltage also affects the transformer’s turns ratio, which determines the voltage transformation between the primary and secondary windings. The turns ratio is calculated by dividing the number of turns in the primary winding by the number of turns in the secondary winding.
For example, if a transformer has a primary voltage of 480V and a secondary voltage of 120V, the turns ratio would be 4:1 (480V ÷ 120V). This means that for every four turns in the primary winding, there is one turn in the secondary winding.
Calculate KVA Rating
To calculate the kVA rating of a transformer, first determine the total wattage of all devices powered by the transformer. Divide the total wattage by the output voltage to obtain the total amperage. Multiply the total amperage by the output voltage, then divide the result by 1000. This calculation yields the kVA rating.
Consider potential future growth in load requirements when selecting a transformer. Add a safety margin of 20-50% to the calculated kVA to ensure the transformer can handle increased demand.
Accounting for special load types
Linear loads, including incandescent lighting and heating elements, draw current in proportion to the applied voltage. In contrast, non-linear loads, such as electronic devices and variable frequency drives, draw current in a non-sinusoidal manner, generating harmonics that are multiples of the fundamental frequency.
Harmonics cause additional heat and stress on the transformer, necessitating oversizing to accommodate the increased thermal load. The total harmonic distortion (THD) percentage determines the appropriate derating factor, with higher THD levels requiring a larger transformer.
Choose transformer type and cooling method
Dry-type transformers are air-cooled, making them suitable for indoor installations where fire safety is a concern. They have lower maintenance requirements and environmental risks compared to liquid-filled transformers. However, their capacity and efficiency are limited.
Liquid-filled transformers, cooled with oil or less-flammable fluids, provide higher efficiency and capacity ratings. They are commonly used for outdoor installations and heavy-duty applications. The cooling fluid dissipates heat more effectively, enabling more compact designs. Liquid-filled transformers require more maintenance and pose greater environmental risks in case of leaks.
FAQs
What is the difference between a transformer’s kVA rating and its power rating?
A transformer’s kVA rating represents its apparent power capacity, while its power rating (measured in kilowatts) represents the actual power it can deliver to the load.
What is the impact of undersizing or oversizing a transformer?
Undersizing a transformer can lead to overheating, reduced lifespan, and potential safety hazards. Oversizing a transformer can result in increased costs and reduced efficiency.
What are the consequences of improper transformer sizing?
Improper transformer sizing can lead to power quality issues, reduced equipment lifespan, increased energy costs, and potential safety hazards such as overheating and fire risks.
What is the relationship between kVA rating and size of transformer?
The kVA rating of a transformer is directly proportional to its physical size. As the kVA rating increases, the transformer’s dimensions, including its core, windings, and overall volume, must also increase to accommodate the higher power capacity. Larger kVA ratings require more materials and result in bigger, heavier transformers to handle the increased electrical load efficiently and safely.